6. Communication between Molecules
Aede de Groot, Willem Koert
When two molecules have to interact like a steroid hormone and its receptor, there have to be instruments that tell the hormone how to find and recognize its receptor. The two molecules have to communicate in one way or the other. They have to feel whether a receptor-ligand complex can be formed or not. In chemical terms we say that the molecules must be able to have a good interaction with each other, which will result in bonding between the steroid and its receptor. In this chapter the interactions that play a role on a molecular level between molecules, is discussed.
The most important interactions are dipole-dipole interactions, apolar interactions, hydrogen bonding and ion interactions. The background of all these interactions will be explained first but a combination of all interactions will finally lead to bonding between the steroid and its receptor.
For a good understanding of interactions on a molecular level it is necessary to go back to the simple carbon compounds of chapter 2. In that chapter the shape of molecules has been discussed, it now will be necessary to know also something about the kind of bonding in these molecules and about the electrical charge distribution in chemical bonds.
Molecules are built up from atoms. Atoms consist of an atom nucleus with positively charged protons and neutral neutrons. Protons and neutrons have approximately the same mass and more than 99% of the mass of the atom is concentrated in the nucleus. The nucleus is surrounded by negatively charged electrons, which circulate around the nucleus with unmeasurable speed and on a relatively large distance. The number of protons and electrons in an atom is equal, which means that an atom as a whole is neutral, it has no overall charge. The electrons around the nucleus take care of the bonding between atoms in molecules.
The bonds in organic molecules are covalent bonds. This means that bonding is the result of a common bonding electron pair in between the two atoms. Each atom may contribute one electron to this bonding electron pair. In bonds between two similar atoms like the C-C bond in ethane, this bonding electron pair is located in the middle of the bond, in between the two positively charged atomic nuclei.
In bonds between two different atoms, the atom with the largest number of positively charged protons in its nucleus attracts the negatively charged electron pair a bit more to that side. A larger number of protons in the nucleus make an atom more electronegative, which means that such an atom is a stronger attractor of the negatively charged electrons in the bond.
In steroids we have to deal mainly with C-C bonds, with C-H bonds, and with C-O bonds, and sometimes with C-N bonds or C-F bonds. The electronegativity of the atoms in these bonds increases in the direction, which is indicated, with fluor as the most electronegative atom.
In a C-H bond the electrons in the bond are a bit more attracted to the more electronegative C-atom and consequently this side of the bond will become slightly negatively charged, which is indicated with the sign δ-. The H-atom will become a bit positive, which is indicated with the sign δ+. The result is that this bond will become a small dipole. In a dipole the centres of positive and negative charge do not coincide and as a result the bond will have a positive and a negative charged end. A dipole is indicated with an arrow that points to the negatively charged end. A dipole can be compared with a small magnet with a plus and a minus pole.
An individual bond between two different atoms can have a dipole, but this does not always mean that the whole molecule has a dipole. This depends also on the shape of the molecule. In molecules like methane, ethane and CO2 the individual bonds all have dipoles but as the result of the direction of these bonds and thus of the dipoles, the overall resulting dipole is zero. Molecules that do not have a dipole are called apolar molecules (see Figure 1).
Figure 1
In other molecules with polar individual bonds the overall dipole does not become zero and that kind of molecules are called
polar molecules. This is the case with molecules like water, ethanol and ammonia.
A closer look on polar and apolar interactions between molecules learns that they follow a simple law namely like draws to like. Thus polar molecules like interactions with other polar molecules and apolar molecules like other apolar molecules. In general it is so that molecules or parts of molecules with many CH2 and CH3 groups are apolar, and molecules with many OH-groups or C=O-groups are polar.
For polar molecules it is not difficult to explain the reason for their mutual attraction. The positive side of one molecule is attracted by the negative side of the other molecule and visa versa. This interaction is called a dipole-dipole interaction and it is based on electrostatic interaction between oppositely charged parts of molecules. This interaction is comparable with the attraction between opposite poles of two magnets (see Figure 2).
Figure 2
For apolar molecules the attraction is caused by the so called Van der Waals forces. These are weak intermolecular forces, which rely on temporary local dipole-dipole ineractions that result in a weak overall attraction (see Figure 2).
Van der Waals forces operate between all molecules, which can be deduced from the fact that all compounds ultimately can be made liquid, also gasses like methane and carbondioxide. At sufficiently low temperature, the Van der Waals forces overcome the kinetic energy of the heat movement and the molecules condense to a liquid. The attraction between molecules with many CH2 and CH3 groups relies completely on Van der Waals forces and in such molecules the interaction is also called apolar interaction.
When apolar molecules interact in water, the term hydrophobic interaction is used also. In a water environment, apolar molecules like interaction with each other and not with the surrounding polar water molecules. In the biological environment of the cell, which of course is a water environment, this is an important type of interaction.
Van der Waals forces are interactions between different molecules. These interactions are stronger when the interacting surface of the molecules is larger. A bigger molecule usually has a larger surface. Also the shape of a molecule is important, a linear molecule has a larger surface that a spherical one of equal mass, and therefore the interaction between linear molecules are stronger. A larger contact surface is also important for a better interaction between a steroid and its receptor as will be shown in chapter 7 for the bonding between THG and the androgen receptor.
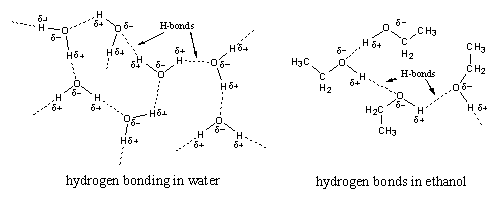
Figure 3
|
The third type of interaction is called a hydrogen bond or H-bond. A hydrogen bond is a stronger type of interaction then the dipole-dipole interaction or the Van der Waals interaction. An H-bond has the strength of about 5% of a normal covalent bond. A hydrogen bond is formed between an H-atom that is bound to an O-atom or an N-atom in one molecule, with an O-atom or an N-atom of another molecule. In this way hydrogen bonds are present in water, alcohols, carbonyl compounds and amides, for instance between peptide bonds (see Figure 3). Weaker hydrogen bonds are formed between amines.
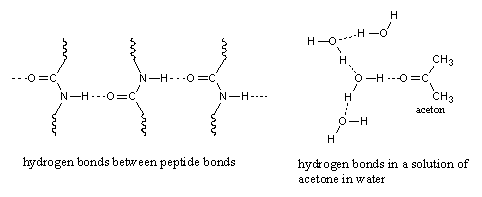
Figure 4
|
A molecule may be a hydrogen bond donor, a hydrogen bond acceptor or both. In water and alcohols the molecules are both, a donor (with the H atom bonded to the O-atom) and an acceptor (with the O-atom).
In a peptide bond the amino group with its H-atoms is only a donor and the O-atom of the carbonyl group is only an acceptor. The latter is clear because a carbonyl group does not have H-atoms that can be donated. A carbonyl group is always just a H-bond acceptor as shown for a solution of acetone in water (see Figure 4).
A fourth type of interaction can be considered as an electrostatic interaction between positively and negatively charged ions. These interactions are also known as ion interactions or salt bridges (see Figure 5).
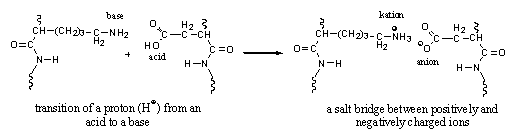
Figure 5
|
An ion is an atom where one electron more or one electron less circulates around the nucleus then the number of protons in the nucleus of that atom. When there is one electron less then the number of protons, the atom as a whole is positively charged and is called a kation. When there is one electron more then the number of protons in the nucleus, the atom will be negatively charged and is called an anion.
The transfer of a positively charged H-atom (a proton, or H+), from an acidic group to a basic group also leads to the formation of negatively and positively charged ions. This process takes place between acidic and basic groups in the side chains of amino acids in proteins. The resulting positive and negative ions will attract each other and form salt bridges (see Figure 5). The formation of salt bridges plays a role in the folding of the chain of amino acids in proteins.
Hydrogen bonds are the main interactions between peptide bonds in the chain of a protein. All types of interactions are operating together between the side chains of the amino acids in a protein. All interactions together lead to folding of the protein chain to a loose ball. All types of interactions are also operating between a protein and other molecules like anabolic steroids with which they form complexes. In chapter 7 we will explain how these interactions result in bonding between steroid hormones and the androgen receptor.
|